
Normal Anatomy and Physiology
The hypothalamus forms the inferior and
lateral walls of the third ventricle, extending from the optic chiasm to the
caudal border of the mamillary bodies (a distance of about 10 mm in the
adult), and is divided into three regions: (1) the supraoptic area dorsal to
the optic chiasm, (2) the central tuberal region, and (3) the caudal
mamillary region. The neurohypophysis consists of a portion of the base of
the hypothalamus, the neural stalk, and the neural (posterior) lobe of the
pituitary gland. The median eminence of the tuber cinereum, while
anatomically part of the neurohypophyseal portion of the hypothalamus, is
involved primarily in the regulation of anterior pituitary function.
The hypothalamus contains small nerve cells
with no distinguishing features, called parvicellular neurons, scattered
throughout its substance; it also contains large neurons, called
magnocellular, which are clustered together to form prominent nuclei. These
magnocellular neurons are located in the paired supraoptic nuclei (SON)
situated above the optic tract and in the paraventricular nuclei (PVN)
immediately beneath the ependyma of the third ventricle. The unmyelinated
nerve fibers from these large cells descend through the infundibulum and
neural stalk to end in the neural lobe of the pituitary gland, forming the
supraopticohypophyseal and paraventriculohypophyseal tracts. The
vasopressinergic and oxytocinergic fibers in the external lamina of the
median eminence appear to arise primarily from the medial parvicellular
group in the PVN. This neuronal system is the probable source of the
vasopressin transported in the portal vessel circulation to the anterior
pituitary. Magnocellular neuronal perikarya that stain for vasopressin
predominate in both the SON and the PVN. However, only scanty oxytocinergic
cell bodies are found in the SON, most being located in the PVN.
In 1982, vasopressin and the opioid peptide
dynorphin were localized to the same magnocellular neurons by
immunohistochemical techniques. Glucagon, cholecystokinin, and angiotensin
II-like immunoreactivities have also been identified in magnocellular
neurosecretory systems, but the functional significance of this coexistence
has not yet been established. The neurohypophyseal hormones are synthesized
as part of a large precursor glycopeptide (propressophysin and
pro-oxyphysin), which also contains carrier proteins (nicotine-sensitive
neurophysin for vasopressin and estrogen-sensitive neurophysin for
oxytocin). These are then transported in secretory vesicles (packets of
hormone and carrier protein) down the axons for storage in the posterior
pituitary gland.
Experimental sectioning of the hypophyseal
stalk results in the accumulation of colloid substance granules varying from
500 to 2000 nm in diameter above the transection, and their disappearance
below it. The stored vesicles in the nerve endings are situated on delicate
membranes that are separated from the basement membranes of adjacent
capillaries by a narrow perivascular space. This anatomic juxtaposition
allows the posterior lobe neurons to act as endocrine organs-that is, they
become cells of the type referred to as neuroendocrine transducers. which
convert neural information to hormonal information. Indeed, the impetus for
release of these stored posterior lobe hormones is an action potential
originating in the neuronal cell body.
The neurons of the neurohypophysis are
primarily regulated by β-adrenergic
(inhibitory) and cholinergic (stimulatory) neurotransmitters.
α-Adrenergic agonists and prostaglandins
of the E group also release ADH. However, magnocellular neurons are also
stimulated by neuropeptides, particularly angiotensin II and endogenous
opioid peptides (endorphins), which may function as physiologic modulators
of posterior pituitary hormone secretion.
Vasopressin
Although it
is now clear that vasopressin has a wide distribution in the central nervous
system, where it may act as a neurotransmitter or neuromodulator in a way
quite unrelated to its posterior pituitary function, its most established
function is its role as antidiuretic hormone (ADH). Following its release
from the posterior pituitary, ADH is transported in the systemic
circulation to the distal renal tubules and collecting ducts, where, after
binding to specific receptors of the V2 type on the cell membrane, it
activates adenylcyclase; this leads to the generation of cyclic adenosine monophosphate (cAMP), which operates as an intracellular second messenger.
The hormonal activity causes a change in the configuration of the luminal
membrane of the cells of the distal renal tubules and collecting ducts,
altering their permeability to water, urea, and possibly sodium. The net
effect of this chain of events is increased reabsorption of water from the
nephron back into the circulation.' Other vasopressin receptors of the V1 (pressor)
type are located on vascular smooth muscle and induce vasoconstriction by
enhancing phosphatidylinositol turnover and increasing the concentration of
free cytosolic calcium.
When plasma
vasopressin levels are under 1 pg/ml, there is maximal free water excretion,
with the urine volume theoretically capable of reaching 20 litres per day,
although in reality a volume in excess of 8 litres per day is rare even in
the absence of detectable circulating vasopressin. When the vasopressin
level is between 1 and 2 pg/ml, its relationship with urine volume is
inverse and exponential, with slight elevations in plasma vasopressin
causing dramatic reductions in urine volume. A plasma vasopressin level of 5
pg/ml or greater results in maximum urine concentration, which can be 1000 mosmol/kg
H2O
if renal function is normal.
Endogenous
prostaglandins (mainly E2) seem to antagonize the effect of
vasopressin-activated cAMP, whereas inhibitors of prostaglandin synthesis
such as indomethacin enhance the effect of vasopressin. Other substances or
conditions that impair the generation or action of vasopressin include hypercalcemia, hypokalemia, and the administration of lithium or the
antibiotic demeclocycline.
This effect
of lithium and demeclocycline accounts for their therapeutic effect in
clinical states of vasopressin "excess," as in the syndrome of inappropriate
ADH secretion (SIADH).
The interplay
between thirst and antidiuretic hormone-the two most important factors in
maintaining fluid balance-is controlled by information received from both
osmoreceptors and volume receptors. The osmoreceptors are situated in the
anterior hypothalamus but appear to be outside the blood-brain barrier.
When the plasma osmolality increases by as little as 1 to 2 percent, the osmoreceptor cells shrink, leading to synthesis of vasopressin and its
release into the systemic circulation. A decrease in osmolality has the
opposite effect. The prime factor
regulating ADH secretion is plasma osmolality. In the event of severe
hypovolemia (a loss of more than 10 to 15 percent of blood volume), the
maintenance of volume takes priority over the maintenance of osmotic
balance. There is some evidence that volume receptors may themselves have a
regulatory effect on osmoreceptor function.
The volume
receptors affecting ADH secretion are located peripherally: in the left
atrium, in the pulmonary veins, and, as stretch receptors, in the arterial
circulation. These receptors respond to changes in volume and arterial
pressure and, through neuronal pathways, lead to an increase or decrease in
ADH secretion to maintain normal volume. There is also a
temperature-dependent oropharyngeal reflex involved in the suppression of
vasopressin. Ingestion of cold water leads to a prompt suppression of plasma
vasopressin even before the water is absorbed from the stomach.
The
renin-angiotensin system is also concerned with the preservation of volume.
If renal perfusion is decreased (e.g., as a result of decreased blood
volume), more of the enzyme renin is released. Renin activates the
conversion of angiotensinogen to angiotensin I (a decapeptide), which in
turn is converted to angiotensin II (an octapeptide). Angiotensin II acts on
the hypothalamus not only to promote ADH secretion but also to increase
thirst, both effects designed to restore volume.
It should be
noted that the hypothalamus contains an independent neuronal renin-angiotensin system, all the components of which arise in situ. It
appears likely that both central and peripheral angiotensin II regulate
thirst and vasopressin secretion, but the relative physiologic importance of
each system has not been established.
A wide
variety of pharmacologic agents affect the release or action of ADH. Thyroid
hormone and cortisol seem to modulate the osmotic set-point for the release
of ADH. Thus, hypothyroidism and hypoadrenocorticism enhance ADH release,
which contributes to the hyponatremia seen in these disorders.
Glucocorticoid excess raises the osmotic threshold for ADH release and
can exacerbate a borderline diabetes insipidus. Drugs that enhance ADH
release include nicotine, chlorpropamide, cholinergic agents, clofibrate,
barbiturates, morphine, anesthetic agents, and carbamazepine. Agents that
decrease the release of ADH include water, ethanol, phenytoin, and
anticholinergic agents.
"Higher"
neural centers have an effect on ADH secretion, as evidenced by the
increased ADH release seen in response to pain, nausea, and stress (both
emotional and physical) and the ability to bring about either diuresis or
antidiuresis experimentally by hypnotic suggestion or psychological
conditioning.
It is of
interest, and perhaps of some clinical importance, that when the
neurohypophyseal system is isolated from the neural input of other parts of
the brain, the system is more active electrically, and ADH secretion is
increased. This hyperfunction of the neurohypophyseal system caused by
isolation or "denervation" may explain in part some of the abnormalities of
ADH secretion seen in some patients with brain injuries.
There is now
much evidence in experimental animals that vasopressin plays an important
role in regulating adrenocorticotropic hormone (ACTH) secretion, acting
directly through V3 receptors on the corticotropes. Vasopressin colocalizes
with corticotropin releasing hormone (CRH), the 41-aminoacid peptide in
the parvicellular neurons of the PVN. Vasopressin potentiates ACTH release
induced by CRH, especially in response to insulin-induced hypoglycemia.
Oxytocin
Oxytocin,
like vasopressin, is a member of the large family of neuropeptides that are
distributed throughout the central nervous system. In extrapituitary
locations, oxytocin probably functions as a neurotransmitter or
neuromodulator; this role is quite separate from its established
physiologic role related to reproduction. The hormone has a selective effect
on the smooth muscle of the uterus and plays a major role in the expulsion
of the fetus and placenta. Labor can begin in a patient with deficient
posterior pituitary function but may be prolonged. It is possible that
other hormonally induced changes in the uterus make it sensitive to normal
concentrations of oxytocin, and that uterine contraction becomes enhanced
only when the proper hormonal priming has taken place.
Oxytocin also
causes contraction of the myoepithelial cells of the breast. These cells
cause the glands to express their contents into ducts so that the milk will
accumulate in the nipples. Once nursing has started, the release of oxytocin
seems to be stimulated via a neural reflex arc that is activated by sensory
nerve endings in the nipple.
Vasopressin
and oxytocin are secreted independent of each other. This fact is
demonstrated in lactating women by two circumstances: (1) The normal
sucking stimulus induces milk letdown without accompanying antidiuresis; and
(2) intravenous hypertonic saline infusion increases ADH production without
producing milk letdown.
Diabetes Insipidus
The
requirements for normal water balance include adequate ADH production,
normal osmoreceptors in the hypothalamus, normally functioning nephrons, a
relatively normal renal medulla such that the osmolar gradient necessary for
water reabsorption can be maintained, a normal thirst center, and the
ability to respond to thirst in an appropriate way. It is clear that the
clinical picture of diabetes insipidus (DI)-polyuria secondary to water
diuresis and polydipsia-may result from dysfunction in any of these areas.
Central
(neurogenic) DI is caused by an insufficient production of ADH in the
hypothalamus. Inability to secrete ADH results in excretion of a dilute
urine. Failure to counter this fluid loss results in dehydration,
hemoconcentration, and hypovolemia. Destruction of the hypothalamic centers
or division of the supraoptic tract above the median eminence causes
permanent DI. Transection below the median eminence, including removal of
the posterior pituitary lobe, causes only a transient polyuria, apparently
because enough ADH can be released from fibers at the level of the median
eminence. Nephrogenic DI (caused by impaired activity of vasopressin at the
level of the kidney) may be the expression of a congenital abnormality or
may result from an acquired disorder (e.g., chronic renal disease,
electrolyte disorders, administration of certain drugs).
DI usually
becomes clinically apparent because of polyuria. The differential diagnosis
of polyuria includes diabetes mellitus, hypercalcuria, psychogenic
polydipsia, chronic renal failure, and the various forms of DI.
Abnormalities of glucose metabolism, electrolyte balance, and renal function
can usually be ruled out with routine laboratory studies. The next step is
to determine whether solute or water excretion is causing the diuresis.
When solute
excretion is responsible for the diuresis, the urinary specific gravity is
usually between 1.010 and 1.035; urine osmolality is usually greater than
300 mosm/kg; and the serum sodium level is variable-for example, it may be
low in hyperglycemia with glucose diuresis or high in subjects dehydrated
by high-protein tube feedings. In mild solute diuresis the urine osmolality
may be high, for example, 800 mosmol/litre; as the rate of solute excretion
increases, however, an increasing amount of water will escape reabsorption
in the proximal tubule and the urine will approach isotonicity. In the
neurosurgical patient population, the major causes of solute diuresis are
osmotic diuretics, hyperglycemia, and corticosteroid
deficiency leading to an inability to retain sodium.
If polyuria
is secondary to water diuresis, as in DI, the urinary specific gravity is
usually between 1.001 and 1.005; urine osmolality is usually between 50 and
150 mosmol/kg; the serum sodium value is generally increased; and thirst is
usually a prominent clinical feature. The common causes of water diuresis
in the neurosurgical patient include (1) DI (Table-1); (2) chronic renal
insufficiency - in which case the patient is usually azotemic with abnormal
renal function studies; (3) nephropathy secondary to multiple myeloma,
amyloidosis, sickle cell disease, radiation nephritis, or systemic lupus
erythematosus or following the relief of obstructive uropathy; (4) the
recovery phase of acute tubular necrosis (perhaps secondary to ischemic or
toxic injury); and (5) fluid overload- a particularly important consideration
in the perioperative period, when the patient's fluid intake is parenterally
administered and not regulated by the thirst mechanism.
Simultaneous
measurement of urine and serum osmolality can provide valuable information.
In all instances of water diuresis the urine is hypoosmolar, but the serum
osmolality is normal or increased in DI and is usually reduced in
compulsive water drinking (Table-2). When the serum is hyperosmolar,
the administration of ADH will differentiate between central DI (there will
be a response of water conservation) and nephrogenic DI (no response to ADH).
Patients with psychogenic polydipsia may be resistant to exogenous
vasopressin because of diminished renal medullary tonicity from chronic
water intake. Compulsive polydipsia is most often secondary to a behavioural
disorder, but the possibility of habitual water drinking secondary to an
organic abnormality of the thirst center should also be considered.
Table-1 Types and Causes of Diabetes Insipidus |
Type |
Cause |
Neurogenic (central) |
Familial;
idiopathic |
Primary Secondary |
Head
trauma; neurosurgical procedures; hypothalamic disease |
Nephrogenic (congenital) |
Unresponsive renal tubules in males (sex-linked) |
Nephrogenic (acquired) |
Electrolyte disorders:
hypercalcemia, hypokalemia. Chronic renal disease Drugs, e.g.. lithium,
demeclocycline. Excess
water intake |
TABLE-2 Abnormalities of Urine and Serum Osmolality and Their Most Common
Causes |
Osmolality Change from Normal |
Urine |
Serum |
Most
Common Cause |
Decreased |
Normal |
Mild or compensated DI,
excess water intake |
Decreased |
Increased |
Any
cause of water diuresis. most
commonly DI |
Decreased |
Decreased |
Psychogenic polydipsia |
Increased |
Increased |
Dehydration |
It should be
kept in mind that hyperosmolality of the serum seen in association with
hyperosmolality of the urine is caused by lack of water intake and not DI.
This situation can be seen in patients with a defective thirst mechanism,
essential hypernatremia, or an impaired level of consciousness such that
they do not recognize their thirst and in those who either have no access to
water or are unable to drink it.
Essential
hypernatremia is a disorder that occurs in the elderly and can be confused
with DI. Despite the elevation of the serum sodium level, there is euvolemia,
normal renal function, and hypodipsia, and the urine is less concentrated
than would be expected from the serum sodium value. There is reduced
vasopressin release in response to the serum osmolality. This condition
occurs in a wide variety of systemic illnesses, but is especially apt to
occur following head trauma and surgery in the hypothalamic area.
In the
immediate postoperative period, a brisk diuresis may occur as a result of
overhydration with isotonic fluids given during surgery. This diuresis can
generally be distinguished from DI by measuring the serum sodium level.
which is usually under 140 meq/litter. However, the diagnosis of mild or
compensated DI can be difficult because the serum osmolality may be
relatively normal despite hypo-osmolar urine. It is in these less
obvious cases that provocative testing is required; a modified dehydration
test is usually adequate. For this test, the patient is deprived of all
fluids until three consecutive hourly urine specimens have the same specific
gravity, usually at 6 to 12 h from the start of testing in patients with
partial DI; sooner in patients with severe DI. The body weight is measured
before the test and hourly during it, and no more than a 3 to 4 percent loss
of body weight is allowed. When the test is terminated, the serum and urine
osmolality are measured simultaneously, 1-deamino-8-D-arginine vasopressin (desmopressin,
DDAVP) is given (1to 2 µg by subcutaneous or intravenous injection or 10
µg by intranasal spray), and the serum and urine osmolality are then
measured hourly for an additional 2 to 4 h. Patients with DI from any cause
generally have an elevated serum osmolality (more than 295 mosmol/kg, and
often much higher) prior to DDAVP administration. In central DI, an increase
in the urine osmolality by at least 50 percent, to reach a level of 600 to
800 mosmol/kg, will occur, whereas in nephrogenic DI, the DDAVP will
increase the urine osmolality by less than 10 percent. In some cases,
measurement of the endogenous plasma vasopressin level and its
responsiveness to dehydration can help to distinguish cases of compulsive
water drinking or nephrogenic DI from central DI.
The clinical
expression of DI in postoperative and post-traumatic situations can be
variable, and the permanence of the DI cannot always be predicted in the
early stages. It is well documented that an occasional patient may require
months or even years to regain an adequate level of ADH function. It should
also be kept in mind that patients who do not have clinically obvious
problems may have a transient episode of DI in response to appropriate
stimuli. Such stimuli may include increased ethanol intake or a significant
increase in corticosteroid dosage.
The clinical
course of DI after head injury or surgery affecting the hypothalamic area
usually follows one of four major patterns:
1. The most
common situation is transient polyuria starting 1 to 3 days after surgery and
lasting 1 to 7 days. This may follow traction on the pituitary stalk, as may
occur during transsphenoidal surgery for a pituitary tumor, and seems to
be an expression of hypothalamic rather than pituitary dysfunction. The
onset and duration of this syndrome are compatible with the onset and
dissolution of local edema.
2. A
triphasic pattern has been described both in humans and in experimental
animals. Polyuria beginning 1 to 2 days after surgery lasts 1 to 7 days and
is followed by a period of normal urinary output lasting up to several
days; abnormally large urinary output then resumes and persists. This triphasic response was produced in experimental animals by transection of
the pituitary stalk and destruction of the hypothalamic median eminence if
the posterior lobe of the pituitary gland was left in place; if the
posterior lobe was removed, there was an immediate and permanent polyuria.
The intermediate phase (also called the interphase) of normal urinary output
seems to be secondary to the release of ADH that was stored in the posterior
pituitary gland; when this ADH has been used up, permanent DI ensues.
3. Polyuria
may begin within the first 2 to 3 postoperative days and be followed by a
small decrease in total urinary volume over the next several days. This is
seen in patients with a partial ADH deficiency that is magnified in the
initial stages because of superimposed local edema.
4. Permanent
polyuria develops within the first 2 to 3 postoperative days and continues
thereafter with no intermediate phase and no significant change in urinary
output volumes. This is seen in patients with immediate, extensive damage to
the hypothalamus. After previously formed ADH has been used up, there is no
recovery of borderline viable cells to produce ADH and modify the
postoperative course.
Treatment of Acute Diabetes Insipidus
The
several patterns of expression of clinical DI mentioned above should be
kept in mind when formulating a postoperative treatment plan.
Therapeutic regimens need to be flexible, because the degree and
duration of the disease process are uncertain.
Patients
with significant preoperative DI seldom have any postoperative
improvement. In that group of patients it is reasonable to resume
preoperative medical treatment as soon as practical.
Patients
likely to develop DI after surgery (i.e., those with surgery in and
around the hypothalamic and pituitary area) are treated expectantly.
Until polyuria becomes apparent, the patient is treated with standard parenteral fluid replacement. Urinary output should be monitored
closely, with volume readings hourly if the patient has an indwelling
bladder catheter, or otherwise as urine specimens are obtained. The
body weight should be recorded before surgery and at least once a day in
the postoperative period. Patients who are able to take liquids by mouth
in the prepolyuria phase are allowed to drink at will, but careful
charting of intake and output must continue.
When
polyuria appears, treatment is determined primarily by the patient's
clinical status, urine volume, and the concentration of the serum
electrolytes (especially sodium) and creatinine. A patient who is
alert and taking food by mouth may be able to regulate the fluid intake
satisfactorily as dictated by thirst, especially if the serum Na+ is 140
meq/litter or less. Body weight and serum electrolytes are measured
twice daily. If the urinary output exceeds an average of 250 ml/h for
two consecutive hours or 3 to 4 litters per day, or if the patient is
unable to maintain adequate intake orally, medication is given.
Intervention is indicated if the serum sodium level rises, especially if
it exceeds 145 meq/litter. DDAVP, given by a subcutaneous, intravenous,
or intranasal route, is the treatment of choice. Aqueous vasopressin,
which has, effects at both the V1 (vascular) and V2 receptors, currently
has little role in the treatment of DI. Pitressin tannate in oil is no
longer manufactured. The most difficult management problem is presented
by patients who are unable to maintain adequate oral intake,
particularly lethargic or obtunded patients. In the latter group, the
clinical parameter of thirst is lost, and meticulous fluid intake and
output records become essential. Twice-daily weight records provide a valuable guide to the state of hydration. If fluid intake and output are
approximately equal, the patient may maintain weight or occasionally
lose a small amount of weight, but will never gain weight. If the
urinary output is very large or there is concern about the patient's
status, the serum electrolytes, blood urea nitrogen (BUN), and
hematocrit are measured twice a day. If the urinary output is relatively
low, once-a-day measurement of these indices is adequate. The serum
osmolality is best measured directly but can be estimated (within 5
percent) by the following formula if the serum electrolytes, BUN, and
blood sugar are known:
Serum osmolality= (2X[Na+]) + (serum glucose/18) + (BUN/3)
Note:sodium in meq/litre, glucose in mg/dl and BUN in mg/dl.
When
blood sugar and renal function are normal, a reliable estimate is twice
the serum sodium value (meq/litter) plus 10. An attempt should be made
to equalize fluid intake with fluid output. The equalization can be done
at intervals varying from 1 h to 1 day, depending on the degree of
polyuria. If the patient has a large urinary output (over 200 ml/h), it
is best to maintain fluid balance on an hourly basis; if the urinary
output is less than that, it is reasonable to maintain fluid balance on
a 4 to 6 h basis.
Because
fluid should be replaced as free water, intravenous fluids should
consist almost solely of dextrose-in-water solutions, and oral fluids
should consist mainly of water, such as tap water. The only electrolyte
replacement required in the postoperative period is what would normally
be given to a postoperative patient; this should be administered as 1
litter of a balanced electrolyte solution and should not be divided in
several infusions.
If salt
solutions are used continuously, they deliver a continuing solute load
to the kidneys, which will aggravate the renal loss of water. Solute
concentration cannot be increased in the absence of ADH. The
administration of saline solutions as replacement for the urinary loss
in diabetes insipidus is probably the most common error in the
management of this problem.
In the
awake patient with an intact thirst mechanism, it is possible to
replace large urinary outputs by oral intake, particularly if some
intravenous supplementation is also used. In the very young or in older
patients with associated medical problems, it is preferable to keep
fluid intake at lower levels. DDAVP can be used at any time in the
postoperative period if there is careful monitoring of fluid balance.
An iatrogenic
inappropriate ADH-like syndrome can be created in patients given excess
exogenous DDAVP or vasopressin along with excess water by mouth or
parenterally. This complication can be avoided through careful management of
the fluid balance and monitoring of the serum sodium level. The two most
important things to remember in managing acute DI are that the primary
problem is water loss and that the process is not static but dynamic.
Diabetes insipidus can be a serious disorder and, if not corrected, can lead
to death.
Treatment of Chronic Diabetes Insipidus
Posterior Pituitary Hormone Preparations
Aqueous
vasopressin is a sterile solution of synthetic 8-arginine vasopressin
available in 0.5- and 1-ml ampoules of 20 IU /ml. The short duration of
action of this preparation (4 to 6 h) and its effects on tissues other than
the kidney make it unsuitable for prolonged use. In the acute stage a slow,
continuous infusion of not more than 3 IU/h or intermittent intramuscular
injections of 0.1 to 1.0 ml are usually effective. However, aqueous
vasopressin can cause angina in patients with coronary artery disease, as
well as nausea and vomiting, through its effects on the splanchnic
vasculature.
Desmopressin acetate (DDAVP) is a long-acting analogue of arginine vasopressin. It is provided in an
aqueous solution in a 10 ml vial in a concentration of 4.0
µg/ml. The
altered chemical structure of DDAVP relative to vasopressin retards its
degradation, and it has high antidiuretic-to-pressor potency (2000:1),
essentially acting only on the V2 receptors. This agent is now the drug of
choice for treating vasopressin-sensitive DI.
DDAVP is
about 10 times more effective when given by a subcutaneous or intravenous
route than when given by intranasal administration. It is generally
administered twice daily by the subcutaneous or intravenous route in doses
of 1 to 4 µg (0.25 to 1 ml), which are equivalent to 4 to 16 IU of arginine
vasopressin. DDAVP is also available for administration by nasal spray or by
rhinal tube. The nasal spray is available in a 5 ml bottle with a spray pump
delivering 50 doses of 10 µg each. If a dose other than 10
µg is
required, the DDAVP may be delivered through a soft plastic rhinal tube
inserted into the nose. The tube has four graduations on it, from 0.05 to
0.2 ml, allowing doses of 5 to 20 µg to be delivered through the nostril.
The DDAVP rhinal tube is provided in a 2.5 ml vial with two applicators. The
concentration of DDAVP in both spray and rhinal tube is 0.1 mg/ml. Of
interest, DDAVP is effective in maintaining homeostasis in mild haemophilia A
and in von Willebrand's disease (type I).
Lysine
vasopressin nasal spray (Diapid) is a synthetic preparation of
vasopressin 8-lysine. It is supplied in a plastic bottle (8 ml) containing
50 USP posterior pituitary pressor units per millilitre. Administration of
one or two sprays up each nostril 3 to 4 times a day may produce good
control of DI. Each spray delivers approximately 2 ID. This agent is mainly
effective in mild cases.
Pitressin
tannate in oil was a water-insoluble compound of vasopressin that was
obtained from animal sources. It was given intramuscularly and was effective
in small doses. This agent had a variable but long duration of action (24 to
72 h). It is no longer manufactured and is of historical interest only.
Nonpituitary Preparations
In recent
years it has become clear that a number of drugs may be effective in
reducing urine volume when given orally. Among these is chlorpropamide,
an oral hypoglycaemic agent that may effectively reduce the polyuria in
hypothalamic but not in nephrogenic DI. The drug seems to act by potentiating the antidiuretic effect of low levels of endogenous ADH. It is
most effective in mild cases, in which the usual dosage is 50 to 250 mg/day.
Antabuse-like symptoms and hypoglycemia occasionally cause problems as
side effects of this drug.
Paradoxically, thiazide diuretics are the only effective drugs for the
treatment of congenital nephrogenic DI. The presence of vasopressin is not
required for action, and the mechanism is probably contraction of the
extracellular fluid volume owing to loss of sodium. Hydrochlorothiazide
should be used in doses of 50 to 100 mg per day. Potassium supplements may
be required. The use of chlorpropamide and hydrochlorothiazide together,
often in only small doses, may be effective in neurogenic DI because of a
synergistic effect. Furthermore, the thiazide diuretic helps to counteract
the hypoglycemia that may be a problem in patients with growth hormone or
ACTH deficiency when they are treated with chlorpropamide alone.
Other agents for the treatment of DI include
carbamazepine, the antineoplastic agents vincristine
and cyclophosphamide, the hypolipidemic agent clofibrate, and the analgesic
acetaminophen. All may be effective in mild DI by effecting the release of
vasopressin and/or enhancing its effect on the kidney. Carbamazepine, in
particular, may potentiate the action of chlorpropamide.
Pharmacologic and Physiologic Interactions with ADH
1.
Diphenylhydantoin. This commonly used agent suppresses the release of both
ADH and insulin. The enhancement of free water clearance seldom leads to a
significant enough water diuresis to be clinically important, but
occasional severe hyperglycaemic episodes have been reported. This drug
should probably not be used in patients with partial or difficult-to-control
DI.
2.
Glucocorticoids. Glucocorticoid administration frequently leads to
the full clinical expression of an underlying ADH deficiency. The DI does
not disappear when the doses of glucocorticoids are decreased, although
there may be a lessening of severity. The effect of high steroid dosages on
patients with trauma affecting the hypothalamus or with surgery in the
hypothalamic-pituitary area may, on the contrary, benefit DI by reducing
local edema.
3. Thyroid
hormone. It should also be pointed out that not only cortisol but also
thyroid hormone affects the secretion of ADH. Patients with severe
hypothyroidism frequently have hyponatremia due to an "inappropriate ADH-like"
syndrome, and thyroid deficiency as well as adrenocortical deficiency may
alleviate the severity of concomitant DI.
Syndrome of Inappropriate Secretion of Antidiuretic Hormone (SIADH)
The existence
of SIADH was initially proposed to explain the occurrence of renal salt
loss and hyponatremia in patients having neither renal nor adrenal disease.
The basis of the syndrome is an overexpansion of extracellular fluid (ECF)
resulting from abnormal secretion of ADH. The primary features of SIADH
remain those described initially:
1.
Hyponatremia with corresponding hypo-osmolality of the serum and
extracellular fluid
2. Continued renal excretion of sodium (>20 meq/litter)
3. Absence of fluid volume depletion (serum creatinine and BUN are usually
low, in contradistinction to hyponatremia from ECF depletion)
4. Greater
osmolality of the urine than is appropriate for the osmolality
of the plasma
5. Normal
renal and normal adrenocortical function
6. Absence of
peripheral edema
7.
Hypouricemia
The syndrome
is characterized by (1) persistence of the hyponatremia despite the
administration of sodium (even if several hundred milliequivalents of sodium
per day is infused, nearly all of it is lost in the urine) and (2) an
abnormal response to a water load: excess water is retained and not promptly
eliminated.
In the
broadest classification, SIADH is a form of dilutional hyponatremia, and the
sine qua non of the syndrome is increased ECF volume. The size of the
increase is usually 3 to 4 litters, so peripheral edema is not present.
Because of the expanded extracellular volume, the glomerular filtration
rate is increased and the renin-angiotensin-aldosterone mechanism is
suppressed, leading to a decrease in the renal reabsorption of sodium.
Atrial natriuretic peptide (ANP), a peptide derived from the heart, is
increased in SIADH and is of much importance in causing the urinary loss of
sodium in the face of hyponatremia that is characteristic of this disorder.
SIADH can
occur in a wide variety of CNS disorders, including encephalitis, stroke,
head trauma, and brain tumors. It has also been described in pulmonary
disorders such as fungal, viral, and bacterial pneumonias. The ADH is
secreted from the neurohypophysis but is no longer under its normal
regulatory influences. It is not clear whether there is an alteration in
osmoreceptor function, whether inappropriate information is being
transmitted centrally from peripheral volume receptors, or whether some
other situation prevails. In some instances, SIADH may result from the
ectopic production of ADH from a tumor such as an oat cell carcinoma of the
bronchus. Since surgical stress, morphine, barbiturates, and anesthetics may
all stimulate ADH secretion, a transient form of SIADH can occur in the
immediate postoperative period.
Symptoms in
SIADH are related to hyponatremia, which becomes a problem primarily
because of hypo-osmolality. Pseudohyponatremia can be seen in association
with conditions such as increased blood glucose, hyperlipidemia, and
administration of mannitol; in these cases the effective osmolality of the
serum is not reduced.
SIADH usually
causes no symptoms until the serum sodium concentration falls below 120 meq/litter,
but if there is progressive retention of water with rapid reduction in serum
sodium, significant symptoms can appear with a serum sodium concentration
of more than 120 meq/litter. Symptoms are often nonspecific and include
anorexia, nausea, irritability, and an accentuation of focal neurological
deficits if there is underlying structural brain damage. If the serum sodium
concentration drops to less than 110 meq/litter, severe neurological
dysfunction due to brain edema (hyponatremic encephalopathy) may occur, with
areflexia, diffuse muscle weakness, seizures, and stupor. The appearance of
symptoms varies greatly from one patient to another and with the rate of
development of the hyponatremia. Hyponatremia that develops rapidly (in
hours) is much more serious than hyponatremia that develops slowly (in
days), since the mechanisms that regulate cell volume may not have time to
act before cerebral edema occurs.
The treatment
of SIADH is determined by the severity of the symptoms. The basis of all
treatment is elimination of excess water and treatment of the underlying
disease. In patients with minor symptoms, restriction of fluid intake to
about 500 ml/day is adequate. A weight loss of 5 to 10 pounds is generally
needed before serum sodium returns to normal. In senile patients or those
with behaviour disorders, in whom it is difficult to control oral fluid
intake, the use of demeclocycline (600 to 1200 mg/day) or lithium carbonate
(600 to 900 mg/day), both of which block the renal tubular response to ADH,
can be helpful. Furosemide, a loop diuretic that facilitates free water
clearance, may also be useful at (40 to 60 mg/day) along with increased oral salt intake.
If symptoms
are acute and severe, the patient's serum osmolality needs to be raised
more quickly. Hypertonic saline infusions along with a loop diuretic will
expedite the water loss; 1000 ml of 3% NaCl or 500 ml of 5% NaCl is infused
over 4 to 6 h. Furosemide, 40 to 60 mg, can be given intravenously at the
beginning of the infusion and may be repeated in 3 to 4 h. Mannitol infusion
can also be used to accelerate the water loss. These emergency measures are
designed to raise the Na + level above 120 meq/litter, at which point more
conservative measures should be used.
The rate at
which the Na+ should be raised has been somewhat controversial because
central pontine myelinolysis (CPM) has occurred when there is rapid
correction of hyponatremia. Overcorrection to the point of hypernatremia
could be
responsible for CPM, rather than the rate of correction. It was recommended
that hypertonic saline be administered to the symptomatic hyponatremic
patient by an infusion pump, the plan being to raise the Na+ by no more
than 1 meq/litter per hour until the patient is alert and seizurefree or the
Na+ has risen by a total of 20 meq/litter, whichever comes first. Others suggested that the rate of increase of the Na+ should not
be greater than 0.5 meq/litter per hour and 25 meq/litter over 48 h.
The
differentiation of SIADH from the hyponatremia seen in salt depletion is
straightforward. None of the signs of hypovolemia, such as decreased blood
pressure, decreased tissue turgor, and elevated BUN, are present in SIADH.
The clinical picture most often confused with SIADH is that of the patient
with an isotonic fluid loss, usually due to the administration of diuretics,
who is treated with hypotonic fluid replacement. In that case the body will
sacrifice the maintenance of tonicity for the sake of preserving
volume-really a form of hypotonic dehydration. Once again, it should be
stressed that the absence of an increase in extracellular volume precludes a
diagnosis of SIADH.
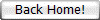
|